- Pulsed DNP
DNP has been used to enhance the sensitivity of MAS NMR at magnetic fields up to 900 MHz/21.1 T/592 GHz and has enabled structural studies of numerous previously inaccessible chemical systems. In these measurements, classical DNP mechanisms, such as the cross-effect, are employed, which rely on continuous, high-power microwave irradiation generated by dedicated sources called gyrotrons. Though this approach is effective, enhancement factors are still well below the theoretical maximum, leaving plenty of room for improvement.
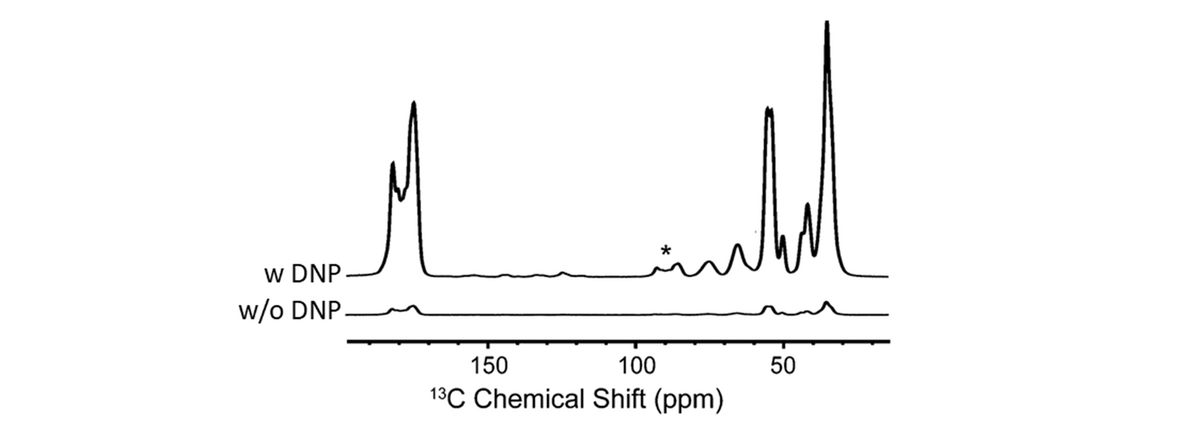
We develop an alternate approach to DNP, in which polarization transfer from electrons to nuclei is not induced by continuous microwave irradiation, but by a well-chosen microwave pulse sequence. For now, we test DNP pulse sequences at low magnetic fields, i.e. at 15 MHz/0.34 T/9.7 GHz (X band) and 51 MHz/1.2 T/34 GHz (Q band), where suitable instrumentation relies on existing technology and can be assembled in house. For the development of DNP pulse sequences, we gain inspiration from the principles and successes of pulsed NMR and EPR spectroscopy.
Pulsed DNP animation
- Photo-DNP
Photo-excitation can, under the right circumstances, produce highly polarized spin systems. A well-known example is the photo-excited triplet state of organic molecules like pentacene. Spin-orbit coupling makes intersystem crossing possible, but not to the same extent for the three magnetic sublevels of the triplet state. As a consequence, and depending on the structure of the organic molecule, the spin polarization of the excited triplet state can become extremely high. In this project, we explore how photo-excitation of various chemical systems is best used to create enhanced nuclear polarization.
- Amorphous calcium carbonate
Amorphous calcium carbonate (ACC) is a precursor phase in the highly controlled, but poorly understood formation of skeletal parts by invertebrate organisms. ACC contains structural water and the formula is approximately CaCO3·H2O. X-ray total scattering and pair distribution function analysis have shown that ACC is truly amorphous and that its short and intermediate range structure differs from the crystalline polymorphs of calcium carbonate. But, in spite of significant attempts, there is, as yet, no good structural model for ACC.
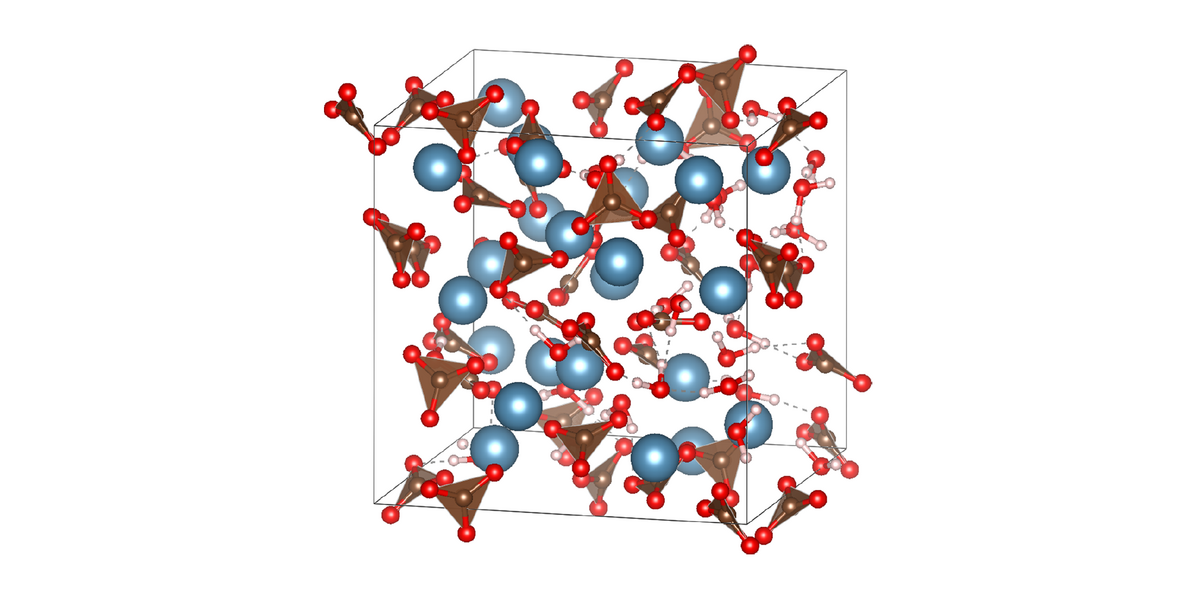
We use MAS NMR to study the structure of ACC. Radiowave pulse sequences selectively reintroduce dipole-dipole couplings (recoupling) while effectively suppressing others (decoupling). Resulting spectra provide information on the chemical environment, internuclear distances, and dynamics of, for example, 1H and 13C nuclei in ACC.
This project is a collaboration with the group of Denis Gebauer, Leibniz Universität Hannover.
- FAT10
The protein FAT10 (human leukocyte antigen-F adjacent transcript 10) plays various roles in the human immune response. Like ubiquitin, FAT10 targets proteins for degradation by the 26S proteasome, but, unlike ubiquitin, it is degraded along with its substrates. FAT10 is loosely folded, which has made it challenging to obtain information about its structure and interactions. So far, it has been possible to determine the structure of a stabilized version of FAT10, in which all cysteine residues had been mutated. Cys-free FAT10 was, however, found to be degraded by the proteasome at a much slower pace than the loosely folded wild-type. Thus, in order to understand the interaction with the proteasome, it is essential to investigate the structure of wild-type FAT10. We do this by MAS NMR.
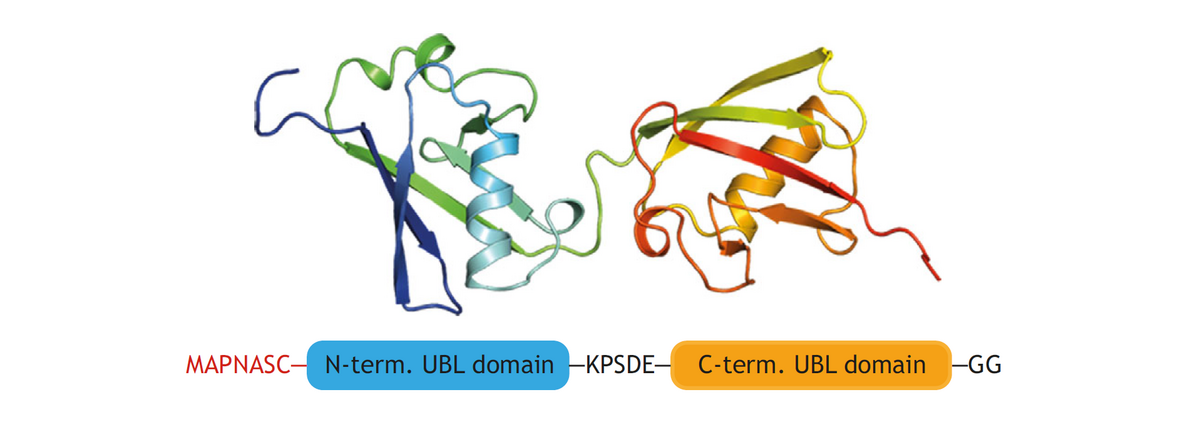
This project is a collaboration with the group of Marcus Groettrup, Department of Biology, Uni Konstanz.
- High-spin iron
Many proteins and enzymes have an iron ion in their active site. EPR spectroscopy can, in principle, provide a wealth of information on the structure of these sites. Unfortunately, if the iron is high-spin, the so-called zero-field-splitting (ZFS) makes it technically challenging to acquire informative spectra. Moreover, the slight structural inhomogeneity, which is naturally present in frozen solutions of proteins, leads to broad distributions of the ZFS parameters, which make spectra difficult to interpret. Together with Mykhailo Azarkh of the Drescher group, we developed a method to extract ZFS distributions from high-frequency EPR spectra, without making assumptions about the underlying structure of the iron site. This allowed us to fully analyze the EPR spectra of the iron-binding protein transferrin and solve the long-standing puzzle of the origin of its remarkable spectral features.